Case Western Reserve University scientist part of team reversing view of how minerals behave deep below our planet’s surface; could apply to other planet studies
Case Western Reserve University scientist James Van Orman is part of an international team that has discovered that minerals deep within our planet may well behave in ways that are the opposite of our previous understandings.
In a paper published this month in the journal Nature, Van Orman and colleagues from France and the California Institute of Technology reported new findings that shed new light on the dynamics of Earth’s deep mantle.
Scientists already know that solid minerals can flow slowly under the high temperatures and pressures of the lower mantle about 400 miles below the surface of the Earth. It is this flow over long periods of time that allows plates to move across the planet’s surface, and into the upper mantle.
It also produces most of the Earth’s volcanoes and earthquakes. That makes it especially important for scientists to have an accurate and reliable understanding of how the mantle flows.
Minerals, ‘taffy’ and timescales
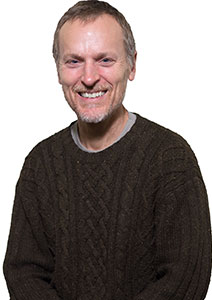
In the new paper, the researchers used numerical modeling to show how the two minerals that make up the lower mantle—bridgmanite and periclase—deform over time.
“It’s pretty important that we now understand the viscosity of the lower mantle at such a granular level,” said Van Orman, a professor of geochemistry and mineral physics. “It means we are in better position to predict how Earth’s mantle convects.”
Convection refers to the stirring of a fluid due to the rise of hotter, less dense material and sinking of colder material. Earth’s deep mantle can behave like a fluid, and convect, because of those two taffy-like minerals.
The new research shows that to model lower mantle viscosity, scientists don’t need to worry about periclase. It’s enough to simply understand how bridgmanite deforms, Van Orman said.
The research was led by scientists at Lille, France, and included work from Van Orman and colleagues at the California Institute of Technology. Funding was provided by the European Research Council and the National Science Foundation.
Earth science basics—and beyond
Earth has three layers: crust, mantle and core. The crust is made of solid rocks and minerals. Beneath the crust is the mantle, which is also composed of solid rocks and minerals, but can flow like a very viscous liquid at high temperatures and pressures. At the center of the Earth is a hot, dense metal core.
The research by Van Orman and the others is focused on the lower mantle, which begins about 416 miles deep and comprises more than half of the Earth’s volume.
Before these new findings, periclase was known as the lower mantle’s soft mineral. In fact, several research teams have succeeded in recent years in deforming bits of bridgmanite and periclase under conditions simulating the conditions of the uppermost lower mantle.
Those experiments showed that periclase is much softer than bridgmanite—but under laboratory conditions.
However, Van Orman said, the experimental samples were deformed much more rapidly than rocks in the lower mantle would deform. It would be impossible to observe the real-time deformation on human timescales, he said.
So, in this new work, the researchers studied the flow of periclase and bridgmanite using numerical modeling and show that while periclase is softer under laboratory conditions—consistent with experimental findings—it becomes much harder than bridgmanite when deformed at lower mantle time scales.
“Imagine a jar of chunky peanut butter. We had thought that periclase was the oil in the peanut butter—so it could make the whole thing softer or harder depending on how the periclase was distributed,” Van Orman said. “But, instead, periclase is like the nuts. No matter how you distribute it, it doesn’t really change the viscosity.”
Therefore, this floating periclase would not change the mantle’s flow behavior, he said.
Applications beyond Earth
If proven true, the assertion could have implications not only for further research and understanding about our own planet, but far beyond.
The researchers suggest their findings could also help astronomers and other scientists better understand the inner workings of newly found exoplanets or other celestial bodies.
“Do Earth-like or super-Earth exoplanets convect or have plate tectonics like our Earth?” Van Orman said. “As we find out more about what’s below us on Earth, it will help us understand what we might find on—or rather, inside—other planets.”
For more information, contact Mike Scott at mike.scott@case.edu.